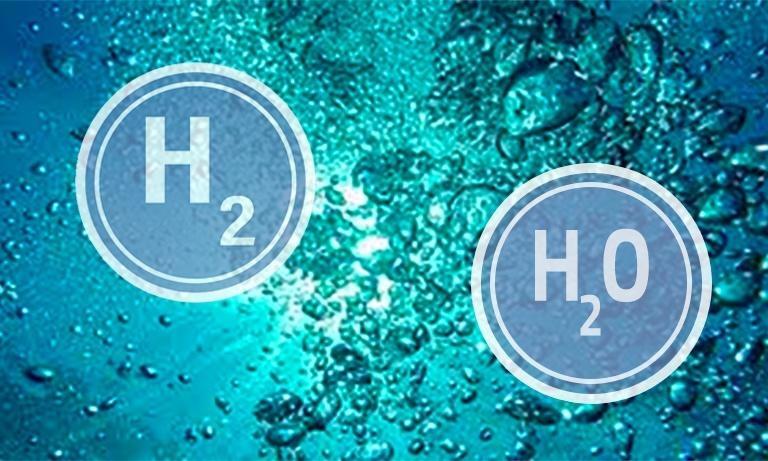
Development perspectives on low-temperature electrolysis
DOI 10.12910/EAI2021-014
by Alfonso Pozio, Francesco Bozza - Batteries and Technologies for Hydrogen Production and Utilization Laboratory; Giuseppe Nigliaccio - Technologies for Districts Urban and Industrial Laboratory - M.Platter – Infrastructures and Services Directorate. Giulia Monteleone – Head of Storage and Use of Energy Division, Energy Storage, Batteries and Technologies for Hydrogen Production and Utilization Laboratory
Water electrolysis is a pivotal technology to boost the hydrogen economy and to decarbonize energy and industry processes. Electrolysers, in addition to the production of hydrogen and oxygen, allow to implement renewable energy storage, through the integration of the electricity grid and the gas network. There are various technologies for water electrolysis, but the most interesting are certainly the membrane one (PEM and AEM) for the possibility to produce hydrogen directly under pressure: a fundamental aspect to favorite the use of hydrogen as energy vector and storage system. ENEA collaborates with Industry, Universities, Research Centres to promote the development and marketing of innovative electrolysers.
L’elettrolisi dell’acqua è una tecnologia fondamentale per favorire la crescita di un’economia idrogeno e per decarbonizzare i settori energetici ed i processi industriali. Gli elettrolizzatori, oltre a produrre idrogeno ed ossigeno, favoriscono la penetrazione delle fonti rinnovabili, attraverso l’integrazione tra la rete elettrica e la rete del gas.Esistono diverse tecnologie di elettrolisi, ma le più interessanti sono quelle a membrana (PEM e AEM) per la possibilità di produrre idrogeno direttamente in pressione: un aspetto fondamentale per favorire l’uso dell’idrogeno come vettore energetico e come “mezzo” di accumulo. ENEA collabora con industria, università ed enti di ricerca per promuovere lo sviluppo commerciale di elettrolizzatori innovativi.
Why the electrolysis? Chemical storage consists in transforming the excess electricity produced by the renewable source into chemical energy contained in a combustible substance. The process that allows this transformation from electricity to chemical energy is precisely electrolysis in which electric current splits water into oxygen and hydrogen. Electrolysis is one of the best known and simplest methods used for the production of pure hydrogen, both on a small and large scale, starting from water, that is, from an extremely abundant primary source. The electrolysis of water occurs when a direct current is passed between two electrodes immersed in an aqueous electrolyte giving rise to the electrolytic decomposition of the water according to the global reaction. 2H2O + electric energy → 2H2↑+ O2↑.
Low temperature electrolysis technologies can be roughly divided according to the type of electrolyte which can be an alkaline liquid electrolyte (AE) or a cationic (PEM) or anionic exchange polymer membrane(AEM) [1]. During electrolysis, the water molecule is broken down to form hydrogen and oxygen. In PEM electrolysis [2, 3], the H+ cations produced at the anode (by the water molecule) move to the cathode through a solid electrolyte, a polymeric acid membrane. H+ cations combine to the cathode forming hydrogen, while oxygen is formed at the anode. In AEM and AE electrolysis occur the same reactions but the charge carrier are the OH- anions moving through a polymeric alkaline membrane or an alkaline solution (Fig.1).
In all the case the membrane (PEM and AEM) or the porous septum (AE) have the function of separating the hydrogen and oxygen produced in the reaction [4]. Several cells of the type shown, connected together and placed in a single container, constitute an electrolyser. The electrodic catalysts will be selected depending on the acidic or alkaline environment. Typically precious metal of platinum group (PMG) are used in the PEM technologies while transition metals as Ni, Co and Fe are used in the AE and AEM systems.
Typical alkaline water electrolysis (AE) operates at a current density of about 400 mA cm–2, at moderate temperatures of 70–90 °C, with a cell voltage in the range 1.85–2.2 V, and conversion efficiencies in the range 50–70%. The advantages of alkaline electrolysis are mainly the followed: not depend upon a noble metal catalyst for the hydrogen production and easily handled due to the relatively low temperatures.
The PEM electrolyzer can operate at a current density of 2000 mA cm–2 at 90 °C, at about 2.1 V. The kinetics of the hydrogen and oxygen production reaction in PEM electrolysis are faster than in alkaline electrolysis due to the acidic nature of the electrolyte and the metal surface of the electrodes. PEM electrolysis offers safety due to the absence of caustic electrolyte. One of the advantages of PEM electrolysis is the possibility of using high pressure on the cathode side, while the anode can be operated at atmospheric pressure.
AEM electrolysis: a developing technology
AEM electrolysis is a developing technology. It summarize some advantages of both technologies, low cost catalysts such as liquid alkaline, compactness and high pressures such as polymeric. Many research organizations and universities are actively involved in this research, largely due to its low cost and the high performance it offers. The specifications, advantages, and disadvantages of the different low temperature electrolysis techniques are summarized in Table 1 and 2.
Table 1 – Comparison of main low temperature water electrolysis technologies.
Alkaline |
PEM |
AEM | |
---|---|---|---|
Electrolyte |
20-30% KOH |
Acid membrane |
Alkaline membrane |
Charge carrier |
OH- |
H+ |
OH- |
Temperature (°C) |
65-100 |
70-90 |
50-70 |
Pressure H2 (bar) |
25-30 |
30-80 |
~30 |
Catalyst |
Ni, Co, Fe |
Pt, Ir, Ru |
Ni, Co, Fe |
Current density mA cm-2 |
200-500 |
800-2500 |
200-500 |
Durability (h) |
90.000 |
<20.000 |
NA |
H2 purity (vol%) |
99,3-99,9 |
99,9999 |
99,99 |
Current efficiency |
50-70.8 |
48,5-65,5 |
39,7 |
Production Nm3 h-1 |
1-760 |
0,265-30 |
0,25-1 |
Energy consumption kWh Nm-3 |
<4.5-7.5 |
5.8-7.3 |
5.2-4.8 |
Power (kW) |
2.8-3534 |
1.8-174 |
1.3-4.8 |
System cost (€ kg-1) |
1300-800 |
2000-1200 |
NA |
Technology status |
Mature |
Mature for small scale |
R&D |
Table 2 – Advantages and disadvantages of alkaline, PEM and AEM electrolysis [7].
Alkaline (AE) | PEM | AEM |
---|---|---|
Advantages | ||
Mature technology | Higher performance | Non-noble metal catalyst |
Non-PGM catalyst | Higher voltage efficiencies | Non corrosive electrolyte |
Long term stabilit | Good partial load | Compact cell design |
Low cost | Rapid system response | Low cost |
Megawatt range | Compact cell design | Absence of leaking |
Cost effective | Dynamic operation | High operating pressure |
High operating pressure | ||
Disadvantages | ||
Low current densities | High cost of components | Laboratory stage |
Cross-over of gas | Acidic corrosive components | Low current densities |
Low dynamic | Possible low durability | Durability |
Low operating pressure | Noble metal catalyst | Membrane degradation |
Corrosive liquid electrolyt | Stack below Megawatt range | Excessive catalyst loading |
Research and development activities in ENEA
ENEA's activities on green hydrogen production are addressed to all the three categories of low-temperature electrolysers, mainly concentrating to catalysts production, electrode and membranes materials development, methods of preparation of electrodes and assemblies for PEM and AE electrolysers [5, 6, 7, 8].
Catalysts and membranes characterization activities are carried out in order to optimize the electrochemical aspects of the polymeric devices. Moreover, research are also addressed to the characterization of components for the production of hydrogen under pressure, with and without a differential between anode and cathode. The possibility of using hydrogen as energy storing system or vector requires gas compression [9]. In fact, hydrogen of all fuels is the one with the lowest volumetric energy density (6.8 MJ/L for 700 bar compressed hydrogen compared to 32 MJ/L for gasoline) (Fig. 2).
So, in all the above electrolyser technologies, the hydrogen operating pressure plays a decisive role [10]. The development of a system for the production of hydrogen under pressure, which also allows to increase the energy performance of the device, would allow an improvement in the overall efficiency of the production and storage system. This represents an important milestone and the growth of this technology would constitute a real technological breakthrough, guaranteeing a better penetration of electrolysis technology from renewable sources.
To obtain high pressures, two alternatives are possible: the use of AE coupled to hydrogen compressors and the sizing of polymer electrolysers (PEM and AEM) for massive hydrogen production. Both options are technically feasible but economically and/or energetically not very convenient. Hence the need to improve the technology of PEM and AEM electrolysers.
Operation under pressure requires the use of thicker and stronger membranes and internal gas re-combinators to keep critical concentrations (mainly H2 in O2) below safety thresholds (4 vol.% H2 in O2). Lower gas permeability across the membrane can be achieved by incorporating various fillers within the membrane material, but this normally leads to less conductive materials.
The critical point in this technology is the development of membranes capable of obtaining high current densities, with a mechanical stability such as to withstand high pressures for thousands of hours, ensuring the necessary purity of the hydrogen produced. According to what has been said, the current commercial standard provides for devices that operate with a pressure of 20-30 bar, even if there are manufacturers that have certified devices in their catalog that can work even at higher pressures. If the operating pressure of the electrolyser were to reach values close to 70 bar, hydrogen could be accumulated, eliminating the first stage of gas compression which is the one that consumes the most energy. Furthermore, mechanical compression could be completely eliminated for some applications, such as the introduction of gas into the network, with great plant and energy advantages.
A different approach is that the two electrolytic compartments operate under pressure and without pressure differential. In this case the system exerts equal pressure on both sides of the membrane, which allows the use of thinner membranes.
According to a theoretical analysis, as pressure increases, the volume of gas bubbles developed during electrolysis decreases. This facilitates the transport of water, decreases ohmic losses in the catalytic layer and improves the electrical contact between the layer and the current collector. This in turn facilitates the transport of water, decreases ohmic losses in the catalytic layer and improves the electrical contact between the layer and the current collector.
In addition, by raising the pressure, it is possible to perform electrolysis at temperatures above 100 °C, thus reducing energy consumption due to a decrease in membrane resistance and overvoltage.
Projects and perspectives
The low temperature electrolysis technologies can play a key-role for the entire hydrogen supply chain, since they allow the production of electricity from renewable sources to interact effectively and efficiently for the production of a gaseous energy vector with zero CO2 emissions. This makes it possible to accumulate, in the form envisaged for gaseous fuels, large quantities of energy and for a very long period. In this sense, the role of hydrogen, seen as an electrical energy storage system, is complementary to the role and use of batteries. The hydrogen thus produced can be injected, for low percentages, directly into the natural gas network. Subsequent use, mixed with natural gas, for example in vehicles, can present significant advantages in reducing emissions [12, 13].Furthermore, the hydrogen produced in this way, in combination with CO2, can be used for what are defined as e-fuels; fuels characterized by low CO2 emissions in the production - use cycle and which may be compatible with the users already present. One example is synthetic methane, produced from hydrogen and CO2 and fully compatible with existing natural gas infrastructures.
Actually, AE electrolysis is the most industrially widespread and technologically mature technology. The main applications are aimed at the on-site production of hydrogen for industrial use with plants of up to hundreds of Nm3/h with operating pressures up to a maximum of 25-30 bar. Lately, under the increasing pressure of the use of renewables, the size of these systems has increased and today modules up to 800 Nm3/h are on the market. Major producers of alkaline electrolysers include Nel (Norway), Thyssenkrupp (Germany), McPhy (France), IHT (Switzerland), Hydrogenics (Canada), Teledyne Energy Systems (USA), Asahi Kasei (Japan), Toshiba (Japan). 50% of the world market of this class of electrolysers is in China which is able to produce machines of the AE type at about 200 USD / kW equal to -80% of the cost of the European ones. About this technology, the main objective is to reduce energy consumption to the theoretical limit of 3.6 kW/m3 in order to reduce the cost of hydrogen. The fundamental points to be addressed in the research field are: 1) the cell engineering in such a way as to minimize the separator/electrode gap by reducing the ohmic drop and develop a more compact design, 2) the development of the electrolytic cell (catalytic coatings of the electrodes, increase in electrode area, decrease in degradation). As regards the management of the overall system, it is necessary to improve the dynamic behavior to electrical loads.
As highlighted above, polymer electrolyte electrolysers (PEMs) have several advantages over AE including the possibility of obtaining theoretically much higher hydrogen pressures thanks to the separating polymer membrane [14]. This last point allows to eliminate or reduce expensive compression systems. On the other hand, the high cost of materials as well as the need to use totally deionized water represents a limit to development. Therefore, although they can reach large sizes (eg: 2 MW ITM plant with single modules of 14 Nm3/h in two blocks of 15 modules each) in fact today they are produced mostly for hydrogen production of a few Nm3/h. There are few industrial companies that produce large polymer electrolysers in consideration of the heavy investments necessary for their development: ITM Power Ltd. (UK), Nel (Norway), Proton Energy Systems (USA), Toshiba (Japan).On both technologies, there are industrial companies in Italy operating on sizes of tens of Nm3/h for AEs, which can be modulated up to MW power and a few liters/h for polymers. The main objective in this case is to reduce the costs related to the materials used.
The fundamental points to be addressed in the research are therefore:
- reduction of the load of noble metals (or replacement with non-CRM metals),
- replacement of titanium with less expensive metals and/or suitable protective coatings,
- development of efficient membranes and alternatives to commercial ones but with reduced costs,
- the development of a cell and system engineering to guarantee high pressures without degradation of the system.
Finally, in the prototype or small-scale commercial phase, there are alkaline membrane electrolysers AEM which should summarize some advantages of both technologies, low-cost catalysts such as liquid alkalis, compactness and high pressures such as polymeric ones [15]. Very few Italian companies operate in this sector. Basic research on various aspects is required on this technology:
- development of a mechanically stable and performing anion membrane capable of guaranteeing suitable purity of hydrogen,
- development of anodic and cathode catalysts and of the related membrane coating methods (CCM),
- development of cell and system engineering to guarantee high pressures.
ENEA with the aim of promoting the development and marketing of innovative electrolyser collaborates with Industry, Universities, Research Centres, and is also involved in national project as the Electrical System Research program (RDS) with the financial support of Minister of Economic Development [16]. With regard to membrane for high pressure, ENEA develops research activities relating to the balance of plant of PEM systems; in the past, activities developed on small PEM system have reached pressure of about 60 bar, and with an improvement in energy performance compared to operation at atmospheric pressure [17]. This pressure value represents the target to be able to think of an injection of hydrogen into a gas network without the use of additional compression systems. Starting by these results, recently ENEA develop a proof or concept [18], in collaboration with national electrolysers producers. Last, in order to develop a regional supply chain for hydrogen, various research projects were also launched [19, 20].
Conclusions
The green hydrogen was universally acknowledged with a key-role for the decarbonization of the energy sector since it could be injected, at low percentages, directly into the natural gas network (blending) or directly used in several industrial application or in mobility sector.
The low temperature membrane electrolysers (PEM and AEM) are the most interesting technologies for green hydrogen production from RES, thanks to the characteristics of compactness, ease of use, low maintenance and high-pressure operation.
The high cost of hydrogen production from electrolysis is still due today to the cost of electricity supply and of the technology. For this reason, there is a need to focus on reducing the production cost and increasing the performance and durability of electrolysers, to achieve further cost reductions in hydrogen production. The achievement of cost targets will depend on innovation that will lead to technological improvements and better adaptation for different technologies and system designs in each specific application.
REFERENCES
- A. Pozio, A. Cemmi, “La produzione dell’idrogeno mediante elettrolisi”, La Chimica e l’Industria, 2010, 12, 108-113
- M. Carmo, D.L. Fritz, J. Merge, D. Stolten, “A comprehensive review on PEM water electrolysis”, Int J Hydrogen Energy 2013, 38, 4901-34.
- S.A. Grigoriev, V.I. Porembsky, V.N. Fateev, “Pure hydrogen production by PEMelectrolysis for hydrogen Energy”, Int J Hydrogen Energy 2006, 31, 171-175.
- R. Phillips, C.W. Dunnill, “Zero gap alkaline electrolysis cell design forrenewable energy storage as hydrogen gas”, RSC Adv. 2016, 6, 100643-100651.
- A. Pozio, N. Lisi, L. Della Seta, S. Dolci, C. D’Angelo, “Effect of cobalt deposition on Ni anodes for alkaline membrane water electrolyser”, Materials Chemistry and Physics, 2020, 242, 122537
- F. Bozza, W. Schafbauer, W.A.Meulenberg, N.Bonanos, “Characterization of La0.995Ca0.005NbO4/Ni anode functional layer by electrophoretic deposition in a La0.995Ca0.005NbO4 electrolyte based PCFC”. International Journal of Hydrogen Energy, 2012, 37, 8027.
- A. Pozio, A. Masci, M. Pasquali, “Nickel-TiO2 nanotube anode for photo-electrolysers”, Solar Energy 2016, 136, 590-596
- A. Cemmi, A. Pozio, E. Serra, “Membrane catalizzate per elettrolizzatori a membrana polimerica”, RT/ENEA, RT/2008/6/TER. , ISSN 0393-3016, March 2008
- H. Steeb, W. Seeger, H.A. Oud, “Hysolar: an overview of the German-Saudi Arabian programme on solar hydrogen”, Int J Hydrogen Energy 1994, 19/8, 683-686.
- P. Medina, M. Santarelli, “Analysis of water transport in a high pressure PEM electrolyzer”, Int J Hydrogen Energy 2020, 35, 5173-86.
- A. Sartbaeva, V.L. Kuznetsov, S.A. Wells, P. P. Edwards, “Hydrogen nexus in a sustainable energy future”, Energy & Environmental Science, 2008, 1/1, 1-196.
- Gazzetta ufficiale dell'Unione europea, Parere del Comitato economico e sociale europeo sul tema Mix energetico nel trasporto, (2008/C 162/12)
- G. Nigliaccio, “Miscele idrogeno/metano in motori a combustione interna”, Ecomondo, Rimini, November 2006.
- A. Grigor’ev, M.M. Khaliullin, N.V. Kuleshov, V.N. Fateev. “Electrolysis of water in a system with a solid polymer electrolyte at elevated pressure”, Russian J Electrochemistry 2001, 37, 819-22.
- I. Vincent, D. Bessarabov, “Low cost hydrogen production by anion exchange membrane electrolysis: A review”, Renewable and Sustainable Energy Reviews 2018, 81, 1690-1704
- https://www.enea.it/it/Ricerca_sviluppo/lenergia/ricerca-di-sistema-elettrico
- G. Nigliaccio, M. Platter, "Test dispositivo di elettrolisi dell'acqua ad alta pressione”,RTI/ENEA, RTI/2009/2.5.5/ISER-UTBOL, April 2011.
- https://industria.enea.it/proof-of-concept
- http://www.piugas.enea.it/
- https://e-co2.it/partners/