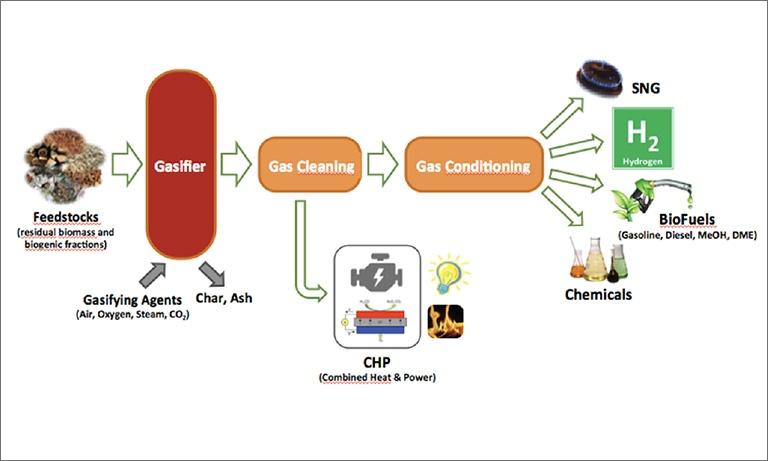
Green hydrogen through biomass gasification
DOI 10.12910/EAI2021-020
by Donatella Barisano, Laboratory of Thermochemical Processes for Biomass, Residues and Waste Valorization
In a future that aims at using hydrogen as an energy carrier to achieve a climate-neutral global economy, its production through biomass gasification can be considered as one of the green options available. However, the exploitation of biomass gasification is not yet ready for large-scale deployment. In this context, ENEA is actively involved in the development and implementation of innovative solutions aimed at achieving the full technological readiness, while ensuring the possibility of producing hydrogen at relatively low-costs. ENEA has been working in the field of biomass gasification for a long time and has acquired substantial expertise in process chemistry and reactor design, with the development of relevant know-how and patents. At ENEA-Trisaia Research Center a technological park has been established with biomass gasification plants based on different reactor setups and sections for gas cleaning and conditioning. Ongoing R&D activities aim at overcoming techno-economic barriers to make biomass gasification reliable and competitive with traditional route, by broadening the flexibility to low-cost, low-value feedstock and introducing innovative and cost-effective methods for gas cleaning and conditioning.
In un futuro che ambisce a utilizzare l'idrogeno come vettore energetico per conseguire l’obiettivo di un'economia globale climaticamente neutra, la sua produzione attraverso la gassificazione della biomassa può essere considerata una delle opzioni green disponibili. Tuttavia, la maturità tecnologica raggiunta non è ancora tale da consentire una sua diffusione su larga scala. In questo contesto, l'ENEA è attivamente impegnata nello sviluppo e nell'implementazione di soluzioni innovative volte al raggiungimento della piena maturità di processo e tecnologica, tale da aprire alla possibilità di produrre idrogeno a costi relativamente contenuti. ENEA opera da tempo nel campo della gassificazione delle biomasse e ha acquisito una notevole esperienza nella chimica di processo e nella progettazione di reattori, con lo sviluppo di proprio know-how e relativi brevetti. Presso il Centro Ricerche ENEA-Trisaia è stato allestito un parco tecnologico con impianti di gassificazione di biomasse basati su reattori di differenti configurazioni e sezioni per la purificazione e il condizionamento del syngas prodotto. Le attività di ricerca e sviluppo in corso puntano a superare le barriere tecnico-economiche per rendere la gassificazione della biomassa affidabile e competitiva con modalità più convenzionali, ampliando la flessibilità a materie prime residuali, di basso costo e di basso valore, e introducendo metodi innovativi ed economici per il trattamento e la conversione del syngas.
In December 2019 the European Union kicked off the Green Deal initiative, the roadmap to a sustainable EU [1]. To achieve such ambitious goals, hydrogen can play an important role and a specific implementation program has been outlined in the communication “A hydrogen strategy for a climate-neutral Europe” [2]. In fact, the use of hydrogen in itself does not emit CO2 and net CO2 emissions can be avoided even in the production phase. Hydrogen from biomass through gasification is one of the options to meet such requirements; biomass is in fact a CO2-neutral energy source being the result of the conversion of solar energy into chemical energy through photosynthesis. At national level this feature has been included in the Italian Strategy for Hydrogen Research [3]. Hydrogen through gasification is included in the roadmap to 2030, with R&D programs at different technology readiness level (TRL) (i.e. Basic research (TRL 2-3), Industrial R&D (TRL 3-5), Demonstration (TRL 5-7)).
The thermochemical gasification of biomass
Gasification is the thermochemical process by which a solid fuel can be converted into a gaseous one of higher value. Following the increased awareness and concerns for global warming, since the 1970s gasification received increasing interest as a process to replace fossil fuels with woody biomass and some categories of biowastes. Such feedstocks are typically residues, locally available, so that gasification enables turning them into a resource with both energy and environmental value.
From a chemical viewpoint, gasification can be seen as an incomplete combustion promoted by an oxidizing agent (e.g. air, pure oxygen, H2O, CO2 and mixtures). Unlike combustion, where heat and an exhaust gas consisting of CO2 and steam are obtained, in gasification the produced gas also contains H2, CO2 and a low amount of CH4. Often called producer gas or syngas, this gas has a calorific value that depends on the adopted gasification agent: low (4-6 MJ/Nm3dry) if produced using air, medium (10-15 MJ/Nm3dry)) if produced with oxygen and/or steam [4]. Low calorific syngas is generally suitable for direct uses aimed for combined heat and power (CHP) production, even on a small-medium scale. Medium calorific syngas is considered of higher value, suitable not only for direct use but also for more innovative process chains, aimed at the production of gas or liquid fuels such as SNG and H2, MeOH, diesel, gasoline, DME, as well as green chemicals (e.g. acetic acid, alcohols, aldehydes) [5]. A general process and applications scheme is shown in Figure 1.
Table 1 shows examples of syngas compositions depending on the gasifying agent and gasification reactor technology.
Table 1 - Illustrative overview of producer gas compositions from biomass.
Gasifying agent | %-v (dry basis) | Gasification Technology | Rerefence | ||||
---|---|---|---|---|---|---|---|
H2 |
O |
CO2 | CH4 | N2 | |||
AIR |
12-20 |
17-22 |
9-15 |
2-3 | 50-54 | downdraft | [6] |
Air |
7-9 |
13-17 |
18-21 |
3 | 50-59 | Bubbling fuidized bed | [7] |
Oxigen |
23 |
36 |
28 |
5 | 7 | Entrained flow | [8] |
Steam/Oxigen |
30-33 |
22-27 | 28-32 | 9-11 | <2 | ICBFB ENEA | [9] |
Steam/Oxigen | 30-31 | 17-19 | 34-35 | 7 | 8-10 | Circulating fluidized bed | [10] |
Steam | 36-42 | 19-24 | 20-25 | 9-12 | - | DFB | [11] |
State of the art
The technology readiness level (TRL) of a gasification process is linked to the final use of the produced syngas. To date, gasification has reached the cogeneration (CHP) market of on small scale (i.e. tens to hundreds kWe). Industrial level can be recognized for CHP application even at medium size level (i.e. few MWe) [12], but there is a small number of plants of this capacity [13]. Lower TRL is obtained in the case of advanced applications, such as high efficiency CHP via SOFC, and the production of liquid and gaseous secondary energy carriers, including hydrogen, where the demonstration or pre-commercial level has been reached so far.
The difference in TRL between small-medium scale CHP and other advanced applications is mainly due to two linked features: economic sustainability and technological solutions for gas purification and conditioning [14].
The first issue is dealing with materials suitable for gasification: in general, the impact of feedstock supply costs is in the range of 20-35% of the operating costs. In order to reduce the impact of this factor, the use of low-cost feedstocks is considered, widening the spectrum to different types, including the renewable fraction of wastes (biowastes) that may eventually provide revenues for their disposal. To achieve these goals, R&D activities on feedstock pre-treatment and on the design of gasification reactors are crucial.
Concerning the second TRL topic, gas cleaning and conditioning can impact investment costs by more than 40% [15,16]. In many relevant applications, the related equipment is shifted from conventional sectors, such as oil industry. These solutions, although effective, achieve economic sustainability on large scales, from several hundred to thousands of MWth at the gasifier input [17-19]. However, achieving full maturity for biomass gasification requires cost-effective operation on smaller scales compared to fossil-based processes. There is a strong need for development of solutions specifically adapted for biomass-derived syngas.
The above constraints indicate a strong need for R&D activities aimed at improving gasification flexibility to feedstocks and achieving significant process intensification to reach economic sustainability even at relatively small scales. Overcoming the above barriers will positively impact on the cost effectiveness of biomass-based conversion processes, making them more consistent with a decentralized production model based on short supply chains, which will likely foster their diffusion.
On these topics, ENEA is among the major players actively engaged in the development and implementation of innovative solutions, with R&D initiatives described in the following sections.
Hydrogen production: conventional vs. gasification methods
H2 from Natural Gas
Of the more than 70 Mton per year of hydrogen produced worldwide, about 75% comes from natural gas (NG) through methane steam reforming (SMR) [20]:
CH4 + 2 H2O → CO2 + 4 H2 (R1)
The SMR reaction is endothermic; the energy required to sustain the process is produced by additional NG. Overall, it is estimated that for every kg of H2 produced via SMR, more than 7 kg of CO2 are emitted [20], corresponding to a molecular ratio of 1 to 1/3, instead of the stoichiometric 1 to 1/4.
To date, the production of H2 from NG is the most economical, with costs ranging from 0.94 US$/kgH2 to 1.78 US$/kgH2. Fuel costs are the largest item, accounting for between 45% and 75% of production costs [21]. These costs are only apparently low because environment impact costs are not included. Higher costs are obtained when solutions to contain the environmental impact of CO2 emissions are also included: in Europe production costs range from 1.73 US$/kgH2 without CO2 capture to 2.32 US$/kgH2 with CCUS (Carbon capture, utilisation and storage), while in China the rates move from 1.78 US$/kgH2 to 2.38 US$/kgH2, respectively [21].
H2 from biomass gasification
Useful feedstocks for green H2 through gasification are both residual lignocellulosic biomass and biogenic fraction of wastes. Residual biomass is derived from forestry, agriculture (e.g. cereal straw, corn stover, pruning), agro-industry (e.g. dried fruit shells, olive pomace, citrus pulp), wood processing, as well as waste streams from other processes that can be valorised for energy purposes rather than be disposed of. Examples are the black liquor from paper mills, or the digestate from Anaerobic Digestion, now facing a problem of overproduction. The biogenic fractions of Municipal Solid Waste can be included too. Referring to the average elemental compositions, for biomass the empirical formula C4H6O3 can be considered. To have an estimation of the maximum potential amount of H2 from biomass, and thus make a comparison with the production from NG, a reaction pathway similar to SMR can be considered:
C4H6O3 + 5 H2O → 4 CO2 + 8 H2 (R2)
R2 points out that the yield of H2 per carbon in the feedstock is, on average, about half of that from NG (R1). However, the lower yield of H2 is balanced by the different nature of CO2 emissions. As known, being biomass the product of photosynthesis, the CO2 from biomass returns to the atmosphere at the end of the process with virtually null overall environment and global warming effects. In the case of SMR, a net increase in CO2 concentration is instead observed. A schematic comparison of the CO2 flow in the two processes is shown in Figure 2.
Research and development activities in ENEA
ENEA has been working in the field of biomass gasification for a long time and has acquired substantial expertise in process chemistry and reactor design, with the development of relevant know-how and patents [22]. At ENEA-Trisaia Research Center a technological park has been established with biomass gasification plants based on different reactor setups (e.g. fixed bed, fluidized bed, rotary kiln, three staged, SCWG) and sections for gas cleaning and conditioning. These plants, available at pilot and laboratory scales, allow to study and to characterize process performances in different applications such as CHP with Internal Combustion Engine and SOFC, or the production of SNG and H2 as secondary energy carriers. Among these, the production of H2 has been studied both within national and EU projects. In the national context, it is worth mentioning the R&D activity carried out within the Electric System Research Programme funded by the Italian Ministry of Economic Development, sub-programme “Bioenergia” [23]. In collaboration with University of L'Aquila, ENEA has studied a process for endogenous H2-enrichment of syngas using SEWGS (sorption-enhanced water-gas shift) sorbents. Thanks to the simultaneous establishment of the WGS reaction and CO2 capture, syngas with high H2 contents were obtained. Since some amount of CH4 was also present in the producer gas, the obtained H2/CH4 mixture turned out to be of interest as a fuel or to produce high purity H2 for more dedicated and advanced applications, after further refining. Produced hydrogen can be mixed with NG or biomethane to produce hydromethane (30% H2 and 70% CH4, by volume) for transport sector or for injection into the national gas distribution grid. In Italy the interest on this approach is led by Snam SpA, which first in Europe, in April 2019 [24] and then in early 2020 [25], injected in its NG distribution grid, at the municipality of Contursi Terme, a mix of H2 and natural gas (H2NG) with an hydrogen content up to 10%v.
In the international context, H2 production from biomass was in particular the goal of the EU project UNIfHY [26]. Coordinated by Guglielmo Marconi University, the project involved the collaboration of nine partners with international excellence on the key features of the proposed approach. The overall performance evaluations were achieved through the integration of the developed components at the Trisaia 1000 kWth pilot plant (Figure 3) and its operation in experimental gasification campaigns coordinated by ENEA.
The gasification plant setup is based on an innovative reactor design, developed for process intensification to achieve reductions in investment and operating costs. Such goals were achieved firstly through a bubbling fluidized bed (BFB) gasifier designed to allow internal recirculation of the bed inventory [27], thus increasing the feedstock residence time in the reaction environment and facilitating its conversion into gas. Secondly, by a bundle of ceramic candles for HT gas filtration directly integrated in the reactor freeboard.
Overall, the UNIfHY project enabled to demonstrate the feasibility of FCV-grade H2 (> 99.99%) through biomass gasification and to provide an estimation of the production costs. The techno-economic assessment indicated production costs of 3-6 €/kgH2 for plants of 10 MWth size [28], thus comparable with that from fossil source (SMR) coupled with CCUS [21, 29].
At present, ENEA is involved in two new EU projects dealing with biomass gasification, i.e. BLAZE [30] and GICO [31]. BLAZE aims at producing a low-contaminant syngas, to feed a 25 kWe SOFC unit. Using syngas in SOFC systems implies the availability of stream with high purity, i.e. concentration levels in the ppm range for most contaminants. To this goal, both primary and secondary methods for gas cleaning and conditioning are included. ENEA is involved in the study of primary methods, investigating low-value active materials, added to the bed inventory, to counteract the contaminants evolvement directly during their formation. Although the main application in BLAZE is different from H2 production, gas purification is a general issue to be faced in biomass gasification for H2 production, as well.
Started only a few months ago, GICO is a multi-purpose project as it provides for different outputs in the context of circular use of resources and sustainability. The reference process is gasification in the presence of calcium-based sorbents for CO2-capture, and its regeneration (calcium looping). This method of feedstock processing will enhance the production of a stream with high H2 concentration from which pure H2 will be more easily obtain later. Moreover, thanks to the action of the sorbent, the process avoids CO2 emissions. On this aspect, ENEA is leading activities on performance evaluation of both natural and innovative materials in fluidized bed and rotary kiln reactors. In order to broaden the spectrum of usable feedstocks, GICO includes the study of the effectiveness of Hydro-Thermal Carbonization (HTC) pre-treatment on gasification performance. Via HTC, the project aims to reduce the impact on production costs by exploiting also low grade feedstocks.
Conclusions
Biomass gasification is a carbon-neutral hydrogen production route that, besides other direct renewable pathways, can contribute to the future green hydrogen production mix. With the establishment of demo plants with different reactor setups, R&D activities at ENEA aim at overcoming techno-economic barriers to make biomass gasification reliable and competitive with conventional route, by broadening the flexibility in terms of feedstock supply (thus accepting low-cost feedstock) and introducing innovative and cost-effective methods for gas cleaning and conditioning.
REFERENCES
- https://ec.europa.eu/info/strategy/priorities-2019-2024/european-green-deal_en
- “A hydrogen strategy for a climate-neutral Europe”, COM/2020/301, July 2020, https://ec.europa.eu/commission/presscorner/detail/en/qanda_20_1257.
- SIRI, Strategia Italiana Ricerca Idrogeno, https://www.miur.gov.it/web/guest/-/prime-linee-guida-per-la-ricerca-italiana-sull-idrogeno.
- Review. Biomass for energy. Tony Bridgwater. J Sci Food Agric 86:1755–1768 (2006)
- Preliminary Screening - Technical and Economic Assessment of Synthesis Gas to Fuels and Chemicals with Emphasis on the Potential for Biomass-Derived Syngas. P.L. Spath and D.C. Dayton. December 2003, NREL/TP-510-34929
- Optimization of the performance of downdraft biomass gasifier installed at National Engineering Research & Development (NERD) Centre of Sri Lanka. Duleeka Gunarathne, Master of Science Thesis EGI 2012: 004 MSC EKV 868. Available at: http://kth.diva-portal.org/smash/get/diva2:494620/FULLTEXT01.pdf
- Gasification of palm empty fruit bunch in a bubbling fluidized bed: A performance and agglomeration study. Pooya Lahijani, Zainal Alimuddin Zainal. Bioresource Technology 102 (2011) 2068–2076
- Oxygen-Blown Entrained Flow Gasification of Biomass: Impact of Fuel Parameters and Oxygen Stoichiometric Ratio. Michael Kremling, Ludwig Briesemeister, Matthias Gaderer, Sebastian Fendt, and Hartmut Spliethoff. Energy Fuels 2017, 31, 3949−3959. DOI: 10.1021/acs.energyfuels.6b02949
- Biomass gasification and in-bed contaminants removal: Performance of iron enriched Olivine and bauxite in a process of steam/O2 gasification. D. Barisano, C. Freda, F. Nanna, E. Fanelli, A. Villone. Bioresource Technology 118 (2012) 187–194
- Steam–oxygen gasification of forest residues and bark followed by hot gas filtration and catalytic reforming of tars: Results of an extended time test Esa Kurkela, Minna Kurkela, Ilkka Hiltunen. Fuel Processing Technology 141 (2016) 148–158
- Steam gasification of various feedstocks at a dual fluidised bed gasifier: Impacts of operation conditions and bed materials. Christoph Pfeifer & Stefan Koppatz & Hermann Hofbauer. Biomass Conv. Bioref. (2011) 1:39–53, DOI 10.1007/s13399-011-0007-1
- http://task33.ieabioenergy.com/content/participants/country_reports.
- http://task33.ieabioenergy.com/menus/show_database
- Biomass Gasification - A synthesis of technical barriers and current research issues for deployment at large scale. DOI: 10.13140/RG.2.1.2593.8406. Report number: f3 2013:5Affiliation: f3 - The Swedish Knowledge Centre for Renewable Transportation Fuels. Available at: https://f3centre.se/app/uploads/f3_report_2013-5_gasification_technology_status_130517.pdf
- Economic assessment of advanced biofuel production via gasification using cost data from the GoBiGas plant. Henrik Thunman1 | Christer Gustavsson2 | Anton Larsson1,2,3 |
- Ingemar Gunnarsson3 | Freddy Tengberg3. Energy Sci Eng. 2019;7:217–229.
- Economy of Biomass-to-Liquids (BTL) plants An engineering assessment. H. Boerrigter. ECN-C--06-019. MAY 2006;
- Production of FT transportation fuels from biomass; technical options, process analysis and optimisation, and development potential. Carlo N. Hamelinck a,, Andre´ P.C. Faaij a, Herman den Uil b, Harold Boerrigter. Energy 29 (2004) 1743–1771.
- Economic assessment of advanced biofuel production via gasification using cost data from the GoBiGas plant. Henrik Thunman1 | Christer Gustavsson2 | Anton Larsson1,2,3 |Ingemar Gunnarsson3 | Freddy Tengberg3. Energy Sci Eng. 2019;7:217–229.
- Assessment of CO2 capture options from various points in steam methane reforming for hydrogen production. R. Soltani, M.A. Rosen, I. Dincer. International journal of hydrogen energy 39 (2014), 20266-20275.
- dato IEA (2018), https://www.iea.org/reports/the-future-of-hydrogen
- http://brevetti.enea.it/elenco.php
- https://www.enea.it/it/Ricerca_sviluppo/lenergia/ricerca-di-sistema-elettrico/accordo-di-programma-MiSE-ENEA-2015-2017/generazione-di-energia-elettrica-con-basse-emissioni-di-carbonio/bioenergia
- https://www.snam.it/en/energy_transition/hydrogen/snam_and_hydrogen/index.html
- https://www.snam.it/it/media/news_eventi/2020/Snam_immissione_sperimentale_idrogeno_Contursi_raddoppiata.html
- https://cordis.europa.eu/project/id/299732
- http://brevetti.enea.it/elenco.php, BREVETTO N. ENEA 657
- https://cordis.europa.eu/docs/results/299/299732/final1-unifhy-final-report-pub.pdf
- Chapter 3 - Hydrogen production. Muhammet Kayfeci, Ali Kec¸ebaş, Mutlucan Bayat. Solar Hydrogen Production, Processes, Systems and Technologies 2019, Pages 45-83. doi.org/10.1016/B978-0-12-814853-2.00003-5
- GA 815284, https://www.blazeproject.eu/
- GA 101006656, https://cordis.europa.eu/project/id/101006656